TOP SCEINCE
Scientists build tiny biological robots from human cells
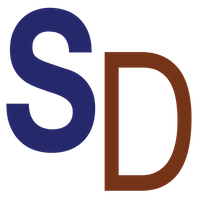
Researchers at Tufts University and Harvard University’s Wyss Institute have created tiny biological robots that they call Anthrobots from human tracheal cells that can move across a surface and have been found to encourage the growth of neurons across a region of damage in a lab dish.
The work follows from earlier research in the laboratories of Michael Levin, Vannevar Bush Professor of Biology at Tufts University School of Arts & Sciences, and Josh Bongard at the University of Vermont in which they created multicellular biological robots from frog embryo cells called Xenobots, capable of navigating passageways, collecting material, recording information, healing themselves from injury, and even replicating for a few cycles on their own. At the time, researchers did not know if these capabilities were dependent on their being derived from an amphibian embryo, or if biobots could be constructed from cells of other species.
In the current study, published in Advanced Science, Levin, along with PhD student Gizem Gumuskaya discovered that bots can in fact be created from adult human cells without any genetic modification and they are demonstrating some capabilities beyond what was observed with the Xenobots. The discovery starts to answer a broader question that the lab has posed — what are the rules that govern how cells assemble and work together in the body, and can the cells be taken out of their natural context and recombined into different “body plans” to carry out other functions by design?
In this case, researchers gave human cells, after decades of quiet life in the trachea, a chance to reboot and find ways of creating new structures and tasks. “We wanted to probe what cells can do besides create default features in the body,” said Gumuskaya, who earned a degree in architecture before coming into biology. “By reprogramming interactions between cells, new multicellular structures can be created, analogous to the way stone and brick can be arranged into different structural elements like walls, archways or columns.” The researchers found that not only could the cells create new multicellular shapes, but they could move in different ways over a surface of human neurons grown in a lab dish and encourage new growth to fill in gaps caused by scratching the layer of cells.
Exactly how the Anthrobots encourage growth of neurons is not yet clear, but the researchers confirmed that neurons grew under the area covered by a clustered assembly of Anthrobots, which they called a “superbot.”
“The cellular assemblies we construct in the lab can have capabilities that go beyond what they do in the body,” said Levin, who also serves as the director of the Allen Discovery Center at Tufts and is an associate faculty member of the Wyss Institute. “It is fascinating and completely unexpected that normal patient tracheal cells, without modifying their DNA, can move on their own and encourage neuron growth across a region of damage,” said Levin. “We’re now looking at how the healing mechanism works, and asking what else these constructs can do.”
The advantages of using human cells include the ability to construct bots from a patient’s own cells to perform therapeutic work without the risk of triggering an immune response or requiring immunosuppressants. They only last a few weeks before breaking down, and so can easily be re-absorbed into the body after their work is done.
In addition, outside of the body, Anthrobots can only survive in very specific laboratory conditions, and there is no risk of exposure or unintended spread outside the lab. Likewise, they do not reproduce, and they have no genetic edits, additions or deletions, so there is no risk of their evolving beyond existing safeguards.
How Are Anthrobots Made?
Each Anthrobot starts out as a single cell, derived from an adult donor. The cells come from the surface of the trachea and are covered with hairlike projections called cilia that wave back and forth. The cilia help the tracheal cells push out tiny particles that find their way into air passages of the lung. We all experience the work of ciliated cells when we take the final step of expelling the particles and excess fluid by coughing or clearing our throats. Earlier studies by others had shown that when the cells are grown in the lab, they spontaneously form tiny multicellular spheres called organoids.
The researchers developed growth conditions that encouraged the cilia to face outward on organoids. Within a few days they started moving around, driven by the cilia acting like oars. They noted different shapes and types of movement — the first. important feature observed of the biorobotics platform. Levin says that if other features could be added to the Anthrobots (for example, contributed by different cells), they could be designed to respond to their environment, and travel to and perform functions in the body, or help build engineered tissues in the lab.
The team, with the help of Simon Garnier at the New Jersey Institute of Technology, characterized the different types of Anthrobots that were produced. They observed that bots fell into a few discrete categories of shape and movement, ranging in size from 30 to 500 micrometers (from the thickness of a human hair to the point of a sharpened pencil), filling an important niche between nanotechnology and larger engineered devices.
Some were spherical and fully covered in cilia, and some were irregular or football shaped with more patchy coverage of cilia, or just covered with cilia on one side. They traveled in straight lines, moved in tight circles, combined those movements, or just sat around and wiggled. The spherical ones fully covered with cilia tended to be wigglers. The Anthrobots with cilia distributed unevenly tended to move forward for longer stretches in straight or curved paths. They usually survived about 45-60 days in laboratory conditions before they naturally biodegraded.
“Anthrobots self-assemble in the lab dish,” said Gumuskaya, who created the Anthrobots. “Unlike Xenobots, they don’t require tweezers or scalpels to give them shape, and we can use adult cells — even cells from elderly patients — instead of embryonic cells. It’s fully scalable — we can produce swarms of these bots in parallel, which is a good start for developing a therapeutic tool.”
Little Healers
Because Levin and Gumuskaya ultimately plan to make Anthrobots with therapeutic applications, they created a lab test to see how the bots might heal wounds. The model involved growing a two-dimensional layer of human neurons, and simply by scratching the layer with a thin metal rod, they created an open ‘wound’ devoid of cells.
To ensure the gap would be exposed to a dense concentration of Anthrobots, they created “superbots” a cluster that naturally forms when the Anthrobots are confined to a small space. The superbots were made up primarily of circlers and wigglers, so they would not wander too far away from the open wound.
Although it might be expected that genetic modifications of Anthrobot cells would be needed to help the bots encourage neural growth, surprisingly the unmodified Anthrobots triggered substantial regrowth, creating a bridge of neurons as thick as the rest of the healthy cells on the plate. Neurons did not grow in the wound where Anthrobots were absent. At least in the simplified 2D world of the lab dish, the Anthrobot assemblies encouraged efficient healing of live neural tissue.
According to the researchers, further development of the bots could lead to other applications, including clearing plaque buildup in the arteries of atherosclerosis patients, repairing spinal cord or retinal nerve damage, recognizing bacteria or cancer cells, or delivering drugs to targeted tissues. The Anthrobots could in theory assist in healing tissues, while also laying down pro-regenerative drugs.
Making New Blueprints, Restoring Old Ones
Gumuskaya explained that cells have the innate ability to self-assemble into larger structures in certain fundamental ways. “The cells can form layers, fold, make spheres, sort and separate themselves by type, fuse together, or even move,” Gumuskaya said. “Two important differences from inanimate bricks are that cells can communicate with each other and create these structures dynamically, and each cell is programmed with many functions, like movement, secretion of molecules, detection of signals and more. We are just figuring out how to combine these elements to create new biological body plans and functions — different than those found in nature.”
Taking advantage of the inherently flexible rules of cellular assembly helps the scientists construct the bots, but it can also help them understand how natural body plans assemble, how the genome and environment work together to create tissues, organs, and limbs, and how to restore them with regenerative treatments.
TOP SCEINCE
Early dark energy could resolve cosmology’s two biggest puzzles
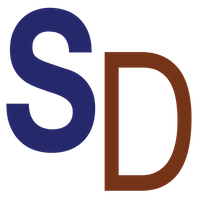
A new study by MIT physicists proposes that a mysterious force known as early dark energy could solve two of the biggest puzzles in cosmology and fill in some major gaps in our understanding of how the early universe evolved.
Now, the MIT team has found that both puzzles could be resolved if the early universe had one extra, fleeting ingredient: early dark energy. Dark energy is an unknown form of energy that physicists suspect is driving the expansion of the universe today. Early dark energy is a similar, hypothetical phenomenon that may have made only a brief appearance, influencing the expansion of the universe in its first moments before disappearing entirely.
Some physicists have suspected that early dark energy could be the key to solving the Hubble tension, as the mysterious force could accelerate the early expansion of the universe by an amount that would resolve the measurement mismatch.
The MIT researchers have now found that early dark energy could also explain the baffling number of bright galaxies that astronomers have observed in the early universe. In their new study, reported in the Monthly Notices of the Royal Astronomical Society, the team modeled the formation of galaxies in the universe’s first few hundred million years. When they incorporated a dark energy component only in that earliest sliver of time, they found the number of galaxies that arose from the primordial environment bloomed to fit astronomers’ observations.
“You have these two looming open-ended puzzles,” says study co-author Rohan Naidu, a postdoc in MIT’s Kavli Institute for Astrophysics and Space Research. “We find that in fact, early dark energy is a very elegant and sparse solution to two of the most pressing problems in cosmology.”
The study’s co-authors include lead author and Kavli postdoc Xuejian (Jacob) Shen, and MIT professor of physics Mark Vogelsberger, along with Michael Boylan-Kolchin at the University of Texas at Austin, and Sandro Tacchella at the University of Cambridge.
Big city lights
Based on standard cosmological and galaxy formation models, the universe should have taken its time spinning up the first galaxies. It would have taken billions of years for primordial gas to coalesce into galaxies as large and bright as the Milky Way.
But in 2023, NASA’s James Webb Space Telescope (JWST) made a startling observation. With an ability to peer farther back in time than any observatory to date, the telescope uncovered a surprising number of bright galaxies as large as the modern Milky Way within the first 500 million years, when the universe was just 3 percent of its current age.
“The bright galaxies that JWST saw would be like seeing a clustering of lights around big cities, whereas theory predicts something like the light around more rural settings like Yellowstone National Park,” Shen says. “And we don’t expect that clustering of light so early on.”
For physicists, the observations imply that there is either something fundamentally wrong with the physics underlying the models or a missing ingredient in the early universe that scientists have not accounted for. The MIT team explored the possibility of the latter, and whether the missing ingredient might be early dark energy.
Physicists have proposed that early dark energy is a sort of antigravitational force that is turned on only at very early times. This force would counteract gravity’s inward pull and accelerate the early expansion of the universe, in a way that would resolve the mismatch in measurements. Early dark energy, therefore, is considered the most likely solution to the Hubble tension.
Galaxy skeleton
The MIT team explored whether early dark energy could also be the key to explaining the unexpected population of large, bright galaxies detected by JWST. In their new study, the physicists considered how early dark energy might affect the early structure of the universe that gave rise to the first galaxies. They focused on the formation of dark matter halos — regions of space where gravity happens to be stronger, and where matter begins to accumulate.
“We believe that dark matter halos are the invisible skeleton of the universe,” Shen explains. “Dark matter structures form first, and then galaxies form within these structures. So, we expect the number of bright galaxies should be proportional to the number of big dark matter halos.”
The team developed an empirical framework for early galaxy formation, which predicts the number, luminosity, and size of galaxies that should form in the early universe, given some measures of “cosmological parameters.” Cosmological parameters are the basic ingredients, or mathematical terms, that describe the evolution of the universe.
Physicists have determined that there are at least six main cosmological parameters, one of which is the Hubble constant — a term that describes the universe’s rate of expansion. Other parameters describe density fluctuations in the primordial soup, immediately after the Big Bang, from which dark matter halos eventually form.
The MIT team reasoned that if early dark energy affects the universe’s early expansion rate, in a way that resolves the Hubble tension, then it could affect the balance of the other cosmological parameters, in a way that might increase the number of bright galaxies that appear at early times. To test their theory, they incorporated a model of early dark energy (the same one that happens to resolve the Hubble tension) into an empirical galaxy formation framework to see how the earliest dark matter structures evolve and give rise to the first galaxies.
“What we show is, the skeletal structure of the early universe is altered in a subtle way where the amplitude of fluctuations goes up, and you get bigger halos, and brighter galaxies that are in place at earlier times, more so than in our more vanilla models,” Naidu says. “It means things were more abundant, and more clustered in the early universe.”
“A priori, I would not have expected the abundance of JWST’s early bright galaxies to have anything to do with early dark energy, but their observation that EDE pushes cosmological parameters in a direction that boosts the early-galaxy abundance is interesting,” says Marc Kamionkowski, professor of theoretical physics at Johns Hopkins University, who was not involved with the study. “I think more work will need to be done to establish a link between early galaxies and EDE, but regardless of how things turn out, it’s a clever — and hopefully ultimately fruitful — thing to try.”
“We demonstrated the potential of early dark energy as a unified solution to the two major issues faced by cosmology. This might be an evidence for its existence if the observational findings of JWST get further consolidated,” Vogelsberger concludes. “In the future, we can incorporate this into large cosmological simulations to see what detailed predictions we get.”
This research was supported, in part, by NASA and the National Science Foundation.
TOP SCEINCE
Plant-derived secondary organic aerosols can act as mediators of plant-plant interactions
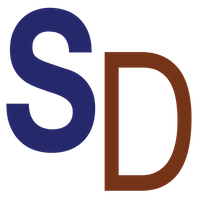
A new study published in Science reveals that plant-derived secondary organic aerosols (SOAs) can act as mediators of plant-plant interactions. This research was conducted through the cooperation of chemical ecologists, plant ecophysiologists and atmospheric physicists at the University of Eastern Finland.
The study showed that Scots pine seedlings, when damaged by large pine weevils, release VOCs that activate defences in nearby plants of the same species. Interestingly, the biological activity persisted after VOCs were oxidized to form SOAs. The results indicated that the elemental composition and quantity of SOAs likely determines their biological functions.
“A key novelty of the study is the finding that plants adopt subtly different defence strategies when receiving signals as VOCs or as SOAs, yet they exhibit similar degrees of resistance to herbivore feeding,” said Professor James Blande, head of the Environmental Ecology Research Group. This observation opens up the possibility that plants have sophisticated sensing systems that enable them to tailor their defences to information derived from different types of chemical cue.
“Considering the formation rate of SOAs from their precursor VOCs, their longer lifetime compared to VOCs, and the atmospheric air mass transport, we expect that the ecologically effective distance for interactions mediated by SOAs is longer than that for plant interactions mediated by VOCs,” said Professor Annele Virtanen, head of the Aerosol Physics Research Group. This could be interpreted as plants being able to detect cues representing close versus distant threats from herbivores.
The study is expected to open up a whole new complex research area to environmental ecologists and their collaborators, which could lead to new insights on the chemical cues structuring interactions between plants.
TOP SCEINCE
Folded or cut, this lithium-sulfur battery keeps going
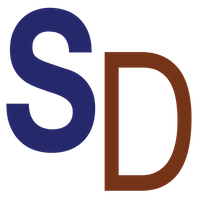
Most rechargeable batteries that power portable devices, such as toys, handheld vacuums and e-bikes, use lithium-ion technology. But these batteries can have short lifetimes and may catch fire when damaged. To address stability and safety issues, researchers reporting in ACS Energy Letters have designed a lithium-sulfur (Li-S) battery that features an improved iron sulfide cathode. One prototype remains highly stable over 300 charge-discharge cycles, and another provides power even after being folded or cut.
The team coated iron sulfide cathodes in different polymers and found in initial electrochemical performance tests that polyacrylic acid (PAA) performed best, retaining the electrode’s discharge capacity after 300 charge-discharge cycles. Next, the researchers incorporated a PAA-coated iron sulfide cathode into a prototype battery design, which also included a carbonate-based electrolyte, a lithium metal foil as an ion source, and a graphite-based anode. They produced and then tested both pouch cell and coin cell battery prototypes.
After more than 100 charge-discharge cycles, Wang and colleagues observed no substantial capacity decay in the pouch cell. Additional experiments showed that the pouch cell still worked after being folded and cut in half. The coin cell retained 72% of its capacity after 300 charge-discharge cycles. They next applied the polymer coating to cathodes made from other metals, creating lithium-molybdenum and lithium-vanadium batteries. These cells also had stable capacity over 300 charge-discharge cycles. Overall, the results indicate that coated cathodes could produce not only safer Li-S batteries with long lifespans, but also efficient batteries with other metal sulfides, according to Wang’s team.
The authors acknowledge funding from the National Natural Science Foundation of China; the Natural Science Foundation of Sichuan, China; and the Beijing National Laboratory for Condensed Matter Physics.
-
Solar Energy3 years ago
DLR testing the use of molten salt in a solar power plant in Portugal
-
Camera1 year ago
DJI Air 3 vs. Mini 4 Pro: which compact drone is best?
-
world news1 year ago
Gulf, France aid Gaza, Russia evacuates citizens
-
Camera1 year ago
Sony a9 III: what you need to know
-
world news1 year ago
Strong majority of Americans support Israel-Hamas hostage deal
-
Camera4 years ago
Charles ‘Chuck’ Geschke, co-founder of Adobe and inventor of the PDF, dies at 81
-
Solar Energy1 year ago
Glencore eyes options on battery recycling project
-
Indian Defense3 years ago
Israeli Radar Company Signs MoU To Cooperate With India’s Alpha Design Technologies