TOP SCEINCE
Durable plastic pollution easily, cleanly degrades with new catalyst
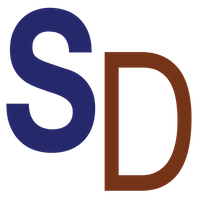
Many people are familiar with the haunting images of wildlife — including sea turtles, dolphins and seals — tangled in abandoned fishing nets.
Now, Northwestern University chemists have developed a new catalyst that quickly, cleanly and completely breaks down Nylon-6 in a matter of minutes — without generating harmful byproducts. Even better: The process does not require toxic solvents, expensive materials or extreme conditions, making it practical for everyday applications.
Not only could this new catalyst play an important role in environmental remediation, it also could perform the first step in upcycling Nylon-6 wastes into higher-value products.
The research will be published on Thursday (Nov. 30) in the journal Chem.
“The whole world is aware of the plastic problem,” said Northwestern’s Tobin Marks, the study’s senior author. “Plastic is a part of our society; we use so much of it. But the problem is: What do we do when we’re finished with it? Ideally, we wouldn’t burn it or put it into landfills. We would recycle it. We’re developing catalysts that deconstruct these polymers, returning them to their original form, so they can be reused.”
Marks is the Charles E. and Emma H. Morrison Professor of Chemistry and Vladimir N. Ipatieff Professor of Catalytic Chemistry at Northwestern’s Weinberg College of Arts and Sciences and a professor of materials science and engineering at Northwestern’s McCormick School of Engineering. He also is a faculty affiliate at the Paula M. Trienens Institute for Sustainability and Energy. Northwestern co-authors include Linda J. Broadbelt, the Sarah Rebecca Roland Professor of Chemical and Biological Engineering and senior associate dean of McCormick, and Yosi Kratish, a research assistant professor in Marks’ group.
A deadly difficulty
From clothing to carpet to seat belts, Nylon-6 is found in a variety of materials that most people use every day. But, when people are done with these materials, they end up in landfills or worse: loose in the environment, including the ocean. According to the World Wildlife Federation, up to 1 million pounds of fishing gear is abandoned in the ocean each year, with fishing nets composed of Nylon-6 making up at least 46% of the Great Pacific Garbage Patch.
“Fishing nets lose quality after a couple years of use,” said Liwei Ye, the paper’s lead first author who is a postdoctoral fellow in Marks’ laboratory. “They become so water-logged that it’s difficult to pull them out of the ocean. And they are so cheap to replace that people just leave them in the water and buy new ones.”
“There is a lot of garbage in the ocean,” Marks added. “Cardboard and food waste biodegrades. Metals sink to the bottom. Then we are left with the plastics.”
The greenest solvent is no solvent
Current methods to dispose of Nylon-6 are limited to simply burying it in landfills. When Nylon-6 is burned, it emits toxic pollutants such as nitrogen oxides, which are linked to various health complications including premature death, or carbon dioxide, an infamously potent greenhouse gas.
Although other laboratories have explored catalysts to degrade Nylon-6, those catalysts require extreme conditions (such as temperatures as high as 350 degrees Celsius), high-pressure steam (which is energetically expensive and inefficient) and/or toxic solvents that only contribute to more pollution.
“You can dissolve plastics in acid, but then you are left with dirty water,” Marks said. “What do you do with that? The goal is always to use a green solvent. And what type of solvent is greener than no solvent at all?”
Recovering building blocks for upcycling
To bypass these issues, the researchers looked to a novel catalyst already developed in Marks’ laboratory. The catalyst harnesses yttrium (an inexpensive Earth-abundant metal) and lanthanide ions. When the team heated Nylon-6 samples to melting temperatures and applied the catalyst without a solvent, the plastic fell apart — reverting to its original building blocks without leaving byproducts behind.
“You can think of a polymer like a necklace or a string of pearls,” Marks explained. “In this analogy, each pearl is a monomer. These monomers are the building blocks. We devised a way to break down the necklace but recover those pearls.”
In experiments, Marks and his team were able to recover 99% of plastics’ original monomers. In principle, those monomers then could be upcycled into higher-value products, which are currently in high demand for their strength and durability.
“Recycled nylon is actually worth more money than regular nylon,” Marks said. “Many high-end fashion brands use recycled nylon in clothes.”
Efficiently targeting Nylon-6
In addition to recovering a high yield of monomers, the catalyst is highly selective — acting only on the Nylon-6 polymers without disrupting surrounding materials. This means industry could apply the catalyst to large volumes of unsorted waste and selectively target Nylon-6.
“If you don’t have a catalyst that’s selective, then how do you separate the nylon from the rest of waste?” Marks said. “You would need to hire humans to sort through all the waste to remove the nylon. That’s enormously expensive and inefficient. But if the catalyst only degrades the nylon and leaves everything else behind, that’s incredibly efficient.”
Recycling these monomers also avoids the need to produce more plastics from scratch.
“These monomers are produced from crude oil, so they have a huge carbon footprint,” Ye said. “That’s just not sustainable.”
What’s next?
After filing a patent for the new process, Marks and his team have already received interest from potential industrial partners. They hope others can use their catalysts on a large scale to help solve the global plastic problem.
“Our research represents a significant step forward in the field of polymer recycling and sustainable materials management,” Ye said. “The innovative approach addresses a critical gap in current recycling technologies, offering a practical and efficient solution for the nylon waste problem. We believe it has implications for reducing the environmental footprint of plastics and contributing to a circular economy.”
The study, “Catalyst metal-ligand design for rapid, selective and solventless depolymerization of Nylon-6 plastics,” was supported by RePLACE (Redesigning Polymers to Leverage A Circular Economy), funded by the Office of Science of the U.S. Department of Energy (award numbers SC0022290 and DE-FG02-03ER15457) and the National Science Foundation (grant number CHE-1856619). Additional support came from the Institute for Catalysis in Energy Processes, which is a major research project within the Center for Catalysis and Surface Science at the Paula M. Trienens Institute for Sustainability and Energy.
TOP SCEINCE
‘Dancing molecules’ heal cartilage damage
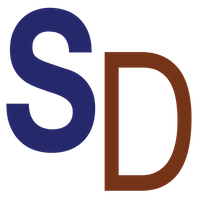
In November 2021, Northwestern University researchers introduced an injectable new therapy, which harnessed fast-moving “dancing molecules,” to repair tissues and reverse paralysis after severe spinal cord injuries.
The researchers also found that, as the molecular motion increased, the treatment’s effectiveness also increased. In other words, the molecules’ “dancing” motions were crucial for triggering the cartilage growth process.
The study was published today (July 26) in the Journal of the American Chemical Society.
“When we first observed therapeutic effects of dancing molecules, we did not see any reason why it should only apply to the spinal cord,” said Northwestern’s Samuel I. Stupp, who led the study. “Now, we observe the effects in two cell types that are completely disconnected from one another — cartilage cells in our joints and neurons in our brain and spinal cord. This makes me more confident that we might have discovered a universal phenomenon. It could apply to many other tissues.”
An expert in regenerative nanomedicine, Stupp is Board of Trustees Professor of Materials Science and Engineering, Chemistry, Medicine and Biomedical Engineering at Northwestern, where he is founding director of the Simpson Querrey Institute for BioNanotechnology and its affiliated center, the Center for Regenerative Nanomedicine. Stupp has appointments in the McCormick School of Engineering, Weinberg College of Arts and Sciences and Feinberg School of Medicine. Shelby Yuan, a graduate student in the Stupp laboratory, was primary author of the study.
Big problem, few solutions
As of 2019, nearly 530 million people around the globe were living with osteoarthritis, according to the World Health Organization. A degenerative disease in which tissues in joints break down over time, osteoarthritis is a common health problem and leading cause of disability.
In patients with severe osteoarthritis, cartilage can wear so thin that joints essentially transform into bone on bone — without a cushion between. Not only is this incredibly painful, patients’ joints also can no longer properly function. At that point, the only effective treatment is a joint replacement surgery, which is expensive and invasive.
“Current treatments aim to slow disease progression or postpone inevitable joint replacement,” Stupp said. “There are no regenerative options because humans do not have an inherent capacity to regenerate cartilage in adulthood.”
What are ‘dancing molecules’?
Stupp and his team posited that “dancing molecules” might encourage the stubborn tissue to regenerate. Previously invented in Stupp’s laboratory, dancing molecules are assemblies that form synthetic nanofibers comprising tens to hundreds of thousands of molecules with potent signals for cells. By tuning their collective motions through their chemical structure, Stupp discovered the moving molecules could rapidly find and properly engage with cellular receptors, which also are in constant motion and extremely crowded on cell membranes.
Once inside the body, the nanofibers mimic the extracellular matrix of the surrounding tissue. By matching the matrix’s structure, mimicking the motion of biological molecules and incorporating bioactive signals for the receptors, the synthetic materials are able to communicate with cells.
“Cellular receptors constantly move around,” Stupp said. “By making our molecules move, ‘dance’ or even leap temporarily out of these structures, known as supramolecular polymers, they are able to connect more effectively with receptors.”
Motion matters
In the new study, Stupp and his team looked to the receptors for a specific protein critical for cartilage formation and maintenance. To target this receptor, the team developed a new circular peptide that mimics the bioactive signal of the protein, which is called transforming growth factor beta-1 (TGFb-1).
Then, the researchers incorporated this peptide into two different molecules that interact to form supramolecular polymers in water, each with the same ability to mimic TGFb-1. The researchers designed one supramolecular polymer with a special structure that enabled its molecules to move more freely within the large assemblies. The other supramolecular polymer, however, restricted molecular movement.
“We wanted to modify the structure in order to compare two systems that differ in the extent of their motion,” Stupp said. “The intensity of supramolecular motion in one is much greater than the motion in the other one.”
Although both polymers mimicked the signal to activate the TGFb-1 receptor, the polymer with rapidly moving molecules was much more effective. In some ways, they were even more effective than the protein that activates the TGFb-1 receptor in nature.
“After three days, the human cells exposed to the long assemblies of more mobile molecules produced greater amounts of the protein components necessary for cartilage regeneration,” Stupp said. “For the production of one of the components in cartilage matrix, known as collagen II, the dancing molecules containing the cyclic peptide that activates the TGF-beta1 receptor were even more effective than the natural protein that has this function in biological systems.”
What’s next?
Stupp’s team is currently testing these systems in animal studies and adding additional signals to create highly bioactive therapies.
“With the success of the study in human cartilage cells, we predict that cartilage regeneration will be greatly enhanced when used in highly translational pre-clinical models,” Stupp said. “It should develop into a novel bioactive material for regeneration of cartilage tissue in joints.”
Stupp’s lab is also testing the ability of dancing molecules to regenerate bone — and already has promising early results, which likely will be published later this year. Simultaneously, he is testing the molecules in human organoids to accelerate the process of discovering and optimizing therapeutic materials.
Stupp’s team also continues to build its case to the Food and Drug Administration, aiming to gain approval for clinical trials to test the therapy for spinal cord repair.
“We are beginning to see the tremendous breadth of conditions that this fundamental discovery on ‘dancing molecules’ could apply to,” Stupp said. “Controlling supramolecular motion through chemical design appears to be a powerful tool to increase efficacy for a range of regenerative therapies.”
TOP SCEINCE
New understanding of fly behavior has potential application in robotics, public safety
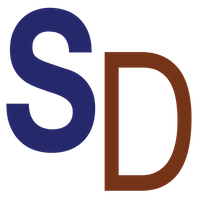
Why do flies buzz around in circles when the air is still? And why does it matter?
“We don’t currently have robotic systems to track odor or chemical plumes,” van Breugel said. “We don’t know how to efficiently find the source of a wind-borne chemical. But insects are remarkably good at tracking chemical plumes, and if we really understood how they do it, maybe we could train inexpensive drones to use a similar process to find the source of chemicals and chemical leaks.”
A fundamental challenge in understanding how insects track chemical plumes — basically, how does the fly find the banana in your kitchen? — is that wind and odors can’t be independently manipulated.
To address this challenge, van Breugel and Stupski used a new approach that makes it possible to remotely control neurons — specifically the “smell” neurons — on the antennae of flying fruit flies by genetically introducing light-sensitive proteins, an approach called optogenetics. These experiments, part of a $450,000 project funded through the Air Force Office of Scientific Research, made it possible to give flies identical virtual smell experiences in different wind conditions.
What van Breugel and Stupski wanted to know: how do flies find an odor when there’s no wind to carry it? This is, after all, likely the wind experience of a fly looking for a banana in your kitchen. The answer is in the Current Biology article, “Wind Gates Olfaction Driven Search States in Free Flight.” The print version will appear in the Sept. 9 issue.
Flies use environmental cues to detect and respond to air currents and wind direction to find their food sources, according to van Breugel. In the presence of wind, those cues trigger an automatic “cast and surge” behavior, in which the fly surges into the wind after encountering a chemical plume (indicating food) and then casts — moves side to side — when it loses the scent. Cast-and-surge behavior long has been understood by scientists but, according to van Breugel, it was fundamentally unknown how insects searched for a scent in still air.
Through their work, van Breugel and Stupski uncovered another automatic behavior, sink and circle, which involves lowering altitude and repetitive, rapid turns in a consistent direction. Flies perform this innate movement consistently and repetitively, even more so than cast-and-surge behavior.
According to van Breugel, the most exciting aspect of this discovery is that it shows flying flies are clearly able to assess the conditions of the wind — its presence, and direction — before deploying a strategy that works well under these conditions. The fact that they can do this is actually quite surprising — can you tell if there is a gentle breeze if you stick your head out of the window of a moving car? Flies aren’t just reacting to an odor with the same preprogrammed response every time like a simple robot, they are responding in context-appropriate manner. This knowledge potentially could be applied to train more sophisticated algorithms for scent-detecting drones to find the source of chemical leaks.
So, the next time you try to swat a fly in your home, consider the fact that flies might actually be a little more aware of some of their natural surroundings than you are. And maybe just open a window to let it out.
TOP SCEINCE
Lampreys possess a ‘jaw-dropping’ evolutionary origin
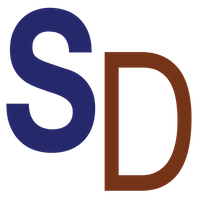
One of just two vertebrates without a jaw, sea lampreys that are wreaking havoc in Midwestern fisheries are simultaneously helping scientists understand the origins of two important stem cells that drove the evolution of vertebrates.
The two cell types — pluripotent blastula cells (or embryonic stem cells) and neural crest cells — are both “pluripotent,” which means they can become all other cell types in the body.
In a new paper, researchers compared lamprey genes to those of the Xenopus, a jawed aquatic frog. Using comparative transcriptomics, the study revealed a strikingly similar pluripotency gene network across jawless and jawed vertebrates, even at the level of transcript abundance for key regulatory factors.
But the researchers also discovered a key difference. While both species’ blastula cells express the pou5 gene, a key stem cell regulator, the gene is not expressed in neural crest stem cells in lampreys. Losing this factor may have limited the ability of neural crest cells to form cell types found in jawed vertebrates (animals with spines) that make up the head and jaw skeleton.
The study will be published July 26 in the journal Nature Ecology & Evolution.
By comparing the biology of jawless and jawed vertebrates, researchers can gain insight into the evolutionary origins of features that define vertebrate animals including humans, how differences in gene expression contribute to key differences in the body plan, and what the common ancestor of all vertebrates looked like.
“Lampreys may hold the key to understanding where we came from,” said Northwestern’s Carole LaBonne, who led the study. “In evolutionary biology, if you want to understand where a feature came from, you can’t look forward to more complex vertebrates that have been evolving independently for 500 million years. You need to look backwards to whatever the most primitive version of the type of animal you’re studying is, which leads us back to hagfish and lampreys — the last living examples of jawless vertebrates.”
An expert in developmental biology, LaBonne is a professor of molecular biosciences in the Weinberg College of Arts and Sciences. She holds the Erastus Otis Haven Chair and is part of the leadership of the National Science Foundation’s (NSF) new Simons National Institute for Theory and Mathematics in Biology.
LaBonne and her colleagues previously demonstrated that the developmental origin of neural crest cells was linked to retaining the gene regulatory network that controls pluripotency in blastula stem cells. In the new study, they explored the evolutionary origin of the links between these two stem cell populations.
“Neural crest stem cells are like an evolutionary Lego set,” said LaBonne. “They become wildly different types of cells, including neurons and muscle, and what all those cell types have in common is a shared developmental origin within the neural crest.”
While blastula stage embryonic stem cells lose their pluripotency and become confined to distinct cell types fairly rapidly as an embryo develops, neural crest cells hold onto the molecular toolkit that controls pluripotency later into development.
LaBonne’s team found a completely intact pluripotency network within lamprey blastula cells, stem cells whose role within jawless vertebrates had been an open question. This implies that blastula and neural crest stem cell populations of jawed and jawless vertebrates co-evolved at the base of vertebrates.
Northwestern postdoctoral fellow and first author Joshua York observed “more similarities than differences” between the lamprey and Xenopus.
“While most of the genes controlling pluripotency are expressed in the lamprey neural crest, the expression of one of these key genes — pou5 — was lost from these cells,” York said. “Amazingly, even though pou5 isn’t expressed in a lamprey’s neural crest, it could promote neural crest formation when we expressed it in frogs, suggesting this gene is part of an ancient pluripotency network that was present in our earliest vertebrate ancestors.”
The experiment also helped them hypothesize that the gene was specifically lost in certain creatures, not something jawed vertebrates developed later on.
“Another remarkable finding of the study is that even though these animals are separated by 500 million years of evolution, there are stringent constraints on expression levels of genes needed to promote pluripotency.” LaBonne said. “The big unanswered question is, why?”
The paper was funded by the National Institutes of Health (grants R01GM116538 and F32DE029113), the NSF (grant 1764421), the Simons Foundation (grant SFARI 597491-RWC) and the Walder Foundation through the Life Sciences Research Foundation. The study is dedicated to the memory of Dr. Joseph Walder.
-
Solar Energy3 years ago
DLR testing the use of molten salt in a solar power plant in Portugal
-
Camera3 years ago
Charles ‘Chuck’ Geschke, co-founder of Adobe and inventor of the PDF, dies at 81
-
world news8 months ago
Gulf, France aid Gaza, Russia evacuates citizens
-
world news3 months ago
Jewish diaspora expresses concern as Iranian drones launch toward Israel
-
Camera3 years ago
80,000MP panoramas: EarthCam announces world’s highest-resolution robotic webcam
-
TOP SCEINCE3 months ago
Can animals count?
-
Solar Energy8 months ago
Glencore eyes options on battery recycling project
-
Camera8 months ago
DJI Air 3 vs. Mini 4 Pro: which compact drone is best?