TOP SCEINCE
Giant viruses found on Greenland ice sheet
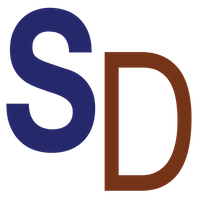
Every spring when the sun rises in the Arctic after months of darkness, life returns. The polar bears pop up from their winter lairs, the arctic tern soar back from their long journey south and the musk oxen wade north.
When the ice blackens it’s ability to reflect the sun diminishes and this accelerates the melting of the ice. Increased melting exacerbates global warming.
But researchers might have found a way to control the snow algae growth — and maybe in the long run reduce some of the ice from melting. Living on the ice alongside the algae, postdoc Laura Perini from the Department of Environmental Science at Aarhus University and her colleagues, have found giant viruses.
She suspects that the viruses feed on the snow algae and could work as a natural control mechanism on the algae blooms.
“We don’t know a lot about the viruses, but I think they could be useful as a way of alleviating ice melting caused by algal blooms. How specific they are and how efficient it would be, we do not know yet. But by exploring them further, we hope to answer some of those questions,” she says.
Bigger than bacteria
Viruses are normally much smaller than bacteria. Regular viruses measure 20-200 nanometers in size, whereas a typical bacteria is 2-3 micrometers. In other words a normal virus is around 1000 times smaller than a bacteria.
That is not the case with giant viruses though.
Giant viruses grow to the size of 2.5 micrometers. That is bigger than most bacteria.
But the giant viruses are not only bigger in size. Their genome is much bigger than regular viruses. Bacteriophages — virus infecting bacteria — have between 100,000 and 200,000 letters in their genome. Giant viruses have around 2,500,000.
Never found on the ice before
Giant viruses were first discovered in 1981, when researchers found them in the ocean. These viruses had specialized in infecting green algae in the sea. Later, giant viruses were found in soil on land and even in humans.
But it’s the first time that giant viruses have been found living on the surface ice and snow dominated by microalgae, Laura Perini explains.
“We analyzed samples from dark ice, red snow and melting holes (cryoconite). In both the dark ice and red snow we found signatures of active giant viruses. And that is the first time they’ve been found on surface ice and snow containing a high abundance of pigmented microalgae.
“A few years ago everyone thought this part of the world to be barren and devoid of life. But today we know that several microorganisms live there — including the giant viruses.”
“There’s a whole ecosystem surrounding the algae. Besides bacteria, filamentous fungi and yeasts, there are protists eating the algae, different species of fungi parasitizing them and the giant viruses that we found, infecting them.
“In order to understand the biological controls acting on the algal blooms, we need to study these last three groups.”
Haven’t seen them with the naked eye
Even though the viruses are giant, they can’t be seen with the naked eye. Laura Perini hasn’t even seen them with a light microscope yet. But she hopes to do so in the future.
“The way we discovered the viruses was by analyzing all the DNA in the samples we took. By sifting through this huge dataset looking for specific marker genes, we found sequences that have high similarity to known giant viruses,” she explains.
To make sure that the viral DNA didn’t come from long dead microorganisms, but from living and active viruses, they also extracted all the mRNA from the sample.
When the sequences of the DNA that form genes are activated, they are transcribed into single stranded pieces called mRNA. These pieces work as recipes for building the proteins the virus needs. If they are present the virus is alive.
“In the total mRNA sequenced from the samples, we found the same markers as in the total DNA, so we know they have been transcribed. It means that the viruses are living and active on the ice,” she says.
DNA and RNA in viruses
At the center of the giant viruses is a cluster of DNA. That DNA contains all the genetic information or recipes needed to create proteins — the chemical compounds that are doing most of the work in the virus.
But in order to use those recipes, the virus needs to transcribe them from double-stranded DNA to single stranded mRNA.
Normal viruses can’t do that. Instead they have strands of RNA floating around in the cell waiting to be activated, when the virus infects an organism and hijacks its cellular production facilities.
Giant viruses can do that themselves which makes them very different from normal viruses.
Whereas DNA from dead viruses can be found in samples, mRNA is broken down much faster. mRNA is therefore an important marker of viral activity. In other words mRNA-recipes of certain proteins show that the viruses are alive and kicking.
Not sure exactly how they work
Because giant viruses are a relatively new discovery not a lot is known about them. In contrast to most other viruses they have a lot of active genes that enable them to repair, replicate, transcribe and translate DNA.
But why that is and exactly what they use it for is not known.
“Which hosts the giant viruses infect, we can’t link exactly. Some of them may be infecting protists while others attack the snow algae. We simply can’t be sure yet,” Laura Perini says.
She’s working hard on discovering more about the giant viruses and has more research coming out soon.
“We keep studying the giant viruses to learn more about their interactions and what is exactly is their role in the ecosystem. Later this year we’ll release another scientific with some more info on giant viruses infecting a cultivated microalgae thriving on the surface ice of the Greenland Ice Sheet,” she concludes.
TOP SCEINCE
‘Dancing molecules’ heal cartilage damage
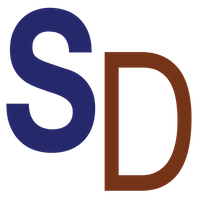
In November 2021, Northwestern University researchers introduced an injectable new therapy, which harnessed fast-moving “dancing molecules,” to repair tissues and reverse paralysis after severe spinal cord injuries.
The researchers also found that, as the molecular motion increased, the treatment’s effectiveness also increased. In other words, the molecules’ “dancing” motions were crucial for triggering the cartilage growth process.
The study was published today (July 26) in the Journal of the American Chemical Society.
“When we first observed therapeutic effects of dancing molecules, we did not see any reason why it should only apply to the spinal cord,” said Northwestern’s Samuel I. Stupp, who led the study. “Now, we observe the effects in two cell types that are completely disconnected from one another — cartilage cells in our joints and neurons in our brain and spinal cord. This makes me more confident that we might have discovered a universal phenomenon. It could apply to many other tissues.”
An expert in regenerative nanomedicine, Stupp is Board of Trustees Professor of Materials Science and Engineering, Chemistry, Medicine and Biomedical Engineering at Northwestern, where he is founding director of the Simpson Querrey Institute for BioNanotechnology and its affiliated center, the Center for Regenerative Nanomedicine. Stupp has appointments in the McCormick School of Engineering, Weinberg College of Arts and Sciences and Feinberg School of Medicine. Shelby Yuan, a graduate student in the Stupp laboratory, was primary author of the study.
Big problem, few solutions
As of 2019, nearly 530 million people around the globe were living with osteoarthritis, according to the World Health Organization. A degenerative disease in which tissues in joints break down over time, osteoarthritis is a common health problem and leading cause of disability.
In patients with severe osteoarthritis, cartilage can wear so thin that joints essentially transform into bone on bone — without a cushion between. Not only is this incredibly painful, patients’ joints also can no longer properly function. At that point, the only effective treatment is a joint replacement surgery, which is expensive and invasive.
“Current treatments aim to slow disease progression or postpone inevitable joint replacement,” Stupp said. “There are no regenerative options because humans do not have an inherent capacity to regenerate cartilage in adulthood.”
What are ‘dancing molecules’?
Stupp and his team posited that “dancing molecules” might encourage the stubborn tissue to regenerate. Previously invented in Stupp’s laboratory, dancing molecules are assemblies that form synthetic nanofibers comprising tens to hundreds of thousands of molecules with potent signals for cells. By tuning their collective motions through their chemical structure, Stupp discovered the moving molecules could rapidly find and properly engage with cellular receptors, which also are in constant motion and extremely crowded on cell membranes.
Once inside the body, the nanofibers mimic the extracellular matrix of the surrounding tissue. By matching the matrix’s structure, mimicking the motion of biological molecules and incorporating bioactive signals for the receptors, the synthetic materials are able to communicate with cells.
“Cellular receptors constantly move around,” Stupp said. “By making our molecules move, ‘dance’ or even leap temporarily out of these structures, known as supramolecular polymers, they are able to connect more effectively with receptors.”
Motion matters
In the new study, Stupp and his team looked to the receptors for a specific protein critical for cartilage formation and maintenance. To target this receptor, the team developed a new circular peptide that mimics the bioactive signal of the protein, which is called transforming growth factor beta-1 (TGFb-1).
Then, the researchers incorporated this peptide into two different molecules that interact to form supramolecular polymers in water, each with the same ability to mimic TGFb-1. The researchers designed one supramolecular polymer with a special structure that enabled its molecules to move more freely within the large assemblies. The other supramolecular polymer, however, restricted molecular movement.
“We wanted to modify the structure in order to compare two systems that differ in the extent of their motion,” Stupp said. “The intensity of supramolecular motion in one is much greater than the motion in the other one.”
Although both polymers mimicked the signal to activate the TGFb-1 receptor, the polymer with rapidly moving molecules was much more effective. In some ways, they were even more effective than the protein that activates the TGFb-1 receptor in nature.
“After three days, the human cells exposed to the long assemblies of more mobile molecules produced greater amounts of the protein components necessary for cartilage regeneration,” Stupp said. “For the production of one of the components in cartilage matrix, known as collagen II, the dancing molecules containing the cyclic peptide that activates the TGF-beta1 receptor were even more effective than the natural protein that has this function in biological systems.”
What’s next?
Stupp’s team is currently testing these systems in animal studies and adding additional signals to create highly bioactive therapies.
“With the success of the study in human cartilage cells, we predict that cartilage regeneration will be greatly enhanced when used in highly translational pre-clinical models,” Stupp said. “It should develop into a novel bioactive material for regeneration of cartilage tissue in joints.”
Stupp’s lab is also testing the ability of dancing molecules to regenerate bone — and already has promising early results, which likely will be published later this year. Simultaneously, he is testing the molecules in human organoids to accelerate the process of discovering and optimizing therapeutic materials.
Stupp’s team also continues to build its case to the Food and Drug Administration, aiming to gain approval for clinical trials to test the therapy for spinal cord repair.
“We are beginning to see the tremendous breadth of conditions that this fundamental discovery on ‘dancing molecules’ could apply to,” Stupp said. “Controlling supramolecular motion through chemical design appears to be a powerful tool to increase efficacy for a range of regenerative therapies.”
TOP SCEINCE
New understanding of fly behavior has potential application in robotics, public safety
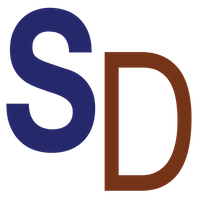
Why do flies buzz around in circles when the air is still? And why does it matter?
“We don’t currently have robotic systems to track odor or chemical plumes,” van Breugel said. “We don’t know how to efficiently find the source of a wind-borne chemical. But insects are remarkably good at tracking chemical plumes, and if we really understood how they do it, maybe we could train inexpensive drones to use a similar process to find the source of chemicals and chemical leaks.”
A fundamental challenge in understanding how insects track chemical plumes — basically, how does the fly find the banana in your kitchen? — is that wind and odors can’t be independently manipulated.
To address this challenge, van Breugel and Stupski used a new approach that makes it possible to remotely control neurons — specifically the “smell” neurons — on the antennae of flying fruit flies by genetically introducing light-sensitive proteins, an approach called optogenetics. These experiments, part of a $450,000 project funded through the Air Force Office of Scientific Research, made it possible to give flies identical virtual smell experiences in different wind conditions.
What van Breugel and Stupski wanted to know: how do flies find an odor when there’s no wind to carry it? This is, after all, likely the wind experience of a fly looking for a banana in your kitchen. The answer is in the Current Biology article, “Wind Gates Olfaction Driven Search States in Free Flight.” The print version will appear in the Sept. 9 issue.
Flies use environmental cues to detect and respond to air currents and wind direction to find their food sources, according to van Breugel. In the presence of wind, those cues trigger an automatic “cast and surge” behavior, in which the fly surges into the wind after encountering a chemical plume (indicating food) and then casts — moves side to side — when it loses the scent. Cast-and-surge behavior long has been understood by scientists but, according to van Breugel, it was fundamentally unknown how insects searched for a scent in still air.
Through their work, van Breugel and Stupski uncovered another automatic behavior, sink and circle, which involves lowering altitude and repetitive, rapid turns in a consistent direction. Flies perform this innate movement consistently and repetitively, even more so than cast-and-surge behavior.
According to van Breugel, the most exciting aspect of this discovery is that it shows flying flies are clearly able to assess the conditions of the wind — its presence, and direction — before deploying a strategy that works well under these conditions. The fact that they can do this is actually quite surprising — can you tell if there is a gentle breeze if you stick your head out of the window of a moving car? Flies aren’t just reacting to an odor with the same preprogrammed response every time like a simple robot, they are responding in context-appropriate manner. This knowledge potentially could be applied to train more sophisticated algorithms for scent-detecting drones to find the source of chemical leaks.
So, the next time you try to swat a fly in your home, consider the fact that flies might actually be a little more aware of some of their natural surroundings than you are. And maybe just open a window to let it out.
TOP SCEINCE
Lampreys possess a ‘jaw-dropping’ evolutionary origin
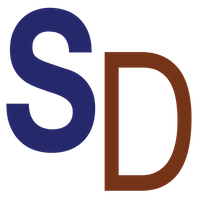
One of just two vertebrates without a jaw, sea lampreys that are wreaking havoc in Midwestern fisheries are simultaneously helping scientists understand the origins of two important stem cells that drove the evolution of vertebrates.
The two cell types — pluripotent blastula cells (or embryonic stem cells) and neural crest cells — are both “pluripotent,” which means they can become all other cell types in the body.
In a new paper, researchers compared lamprey genes to those of the Xenopus, a jawed aquatic frog. Using comparative transcriptomics, the study revealed a strikingly similar pluripotency gene network across jawless and jawed vertebrates, even at the level of transcript abundance for key regulatory factors.
But the researchers also discovered a key difference. While both species’ blastula cells express the pou5 gene, a key stem cell regulator, the gene is not expressed in neural crest stem cells in lampreys. Losing this factor may have limited the ability of neural crest cells to form cell types found in jawed vertebrates (animals with spines) that make up the head and jaw skeleton.
The study will be published July 26 in the journal Nature Ecology & Evolution.
By comparing the biology of jawless and jawed vertebrates, researchers can gain insight into the evolutionary origins of features that define vertebrate animals including humans, how differences in gene expression contribute to key differences in the body plan, and what the common ancestor of all vertebrates looked like.
“Lampreys may hold the key to understanding where we came from,” said Northwestern’s Carole LaBonne, who led the study. “In evolutionary biology, if you want to understand where a feature came from, you can’t look forward to more complex vertebrates that have been evolving independently for 500 million years. You need to look backwards to whatever the most primitive version of the type of animal you’re studying is, which leads us back to hagfish and lampreys — the last living examples of jawless vertebrates.”
An expert in developmental biology, LaBonne is a professor of molecular biosciences in the Weinberg College of Arts and Sciences. She holds the Erastus Otis Haven Chair and is part of the leadership of the National Science Foundation’s (NSF) new Simons National Institute for Theory and Mathematics in Biology.
LaBonne and her colleagues previously demonstrated that the developmental origin of neural crest cells was linked to retaining the gene regulatory network that controls pluripotency in blastula stem cells. In the new study, they explored the evolutionary origin of the links between these two stem cell populations.
“Neural crest stem cells are like an evolutionary Lego set,” said LaBonne. “They become wildly different types of cells, including neurons and muscle, and what all those cell types have in common is a shared developmental origin within the neural crest.”
While blastula stage embryonic stem cells lose their pluripotency and become confined to distinct cell types fairly rapidly as an embryo develops, neural crest cells hold onto the molecular toolkit that controls pluripotency later into development.
LaBonne’s team found a completely intact pluripotency network within lamprey blastula cells, stem cells whose role within jawless vertebrates had been an open question. This implies that blastula and neural crest stem cell populations of jawed and jawless vertebrates co-evolved at the base of vertebrates.
Northwestern postdoctoral fellow and first author Joshua York observed “more similarities than differences” between the lamprey and Xenopus.
“While most of the genes controlling pluripotency are expressed in the lamprey neural crest, the expression of one of these key genes — pou5 — was lost from these cells,” York said. “Amazingly, even though pou5 isn’t expressed in a lamprey’s neural crest, it could promote neural crest formation when we expressed it in frogs, suggesting this gene is part of an ancient pluripotency network that was present in our earliest vertebrate ancestors.”
The experiment also helped them hypothesize that the gene was specifically lost in certain creatures, not something jawed vertebrates developed later on.
“Another remarkable finding of the study is that even though these animals are separated by 500 million years of evolution, there are stringent constraints on expression levels of genes needed to promote pluripotency.” LaBonne said. “The big unanswered question is, why?”
The paper was funded by the National Institutes of Health (grants R01GM116538 and F32DE029113), the NSF (grant 1764421), the Simons Foundation (grant SFARI 597491-RWC) and the Walder Foundation through the Life Sciences Research Foundation. The study is dedicated to the memory of Dr. Joseph Walder.
-
Solar Energy3 years ago
DLR testing the use of molten salt in a solar power plant in Portugal
-
Camera3 years ago
Charles ‘Chuck’ Geschke, co-founder of Adobe and inventor of the PDF, dies at 81
-
world news8 months ago
Gulf, France aid Gaza, Russia evacuates citizens
-
world news3 months ago
Jewish diaspora expresses concern as Iranian drones launch toward Israel
-
Camera3 years ago
80,000MP panoramas: EarthCam announces world’s highest-resolution robotic webcam
-
TOP SCEINCE3 months ago
Can animals count?
-
Solar Energy8 months ago
Glencore eyes options on battery recycling project
-
Camera8 months ago
DJI Air 3 vs. Mini 4 Pro: which compact drone is best?