TOP SCEINCE
Neuroscientists roll out first comprehensive atlas of brain cells: BRAIN initiative consortium takes census of motor cortex cells in mice, marmoset and humans
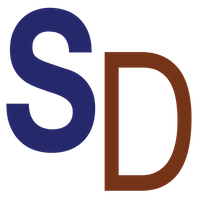
When you clicked to read this story, a band of cells across the top of your brain sent signals down your spine and out to your hand to tell the muscles in your index finger to press down with just the right amount of pressure to activate your mouse or track pad.
The 17 studies, appearing online Oct. 6 in the journal Nature, are the result of five years of work by a huge consortium of researchers supported by the National Institutes of Health’s Brain Research Through Advancing Innovative Neurotechnologies (BRAIN) Initiative to identify the myriad of different cell types in one portion of the brain. It is the first step in a long-term project to generate an atlas of the entire brain to help understand how the neural networks in our head control our body and mind and how they are disrupted in cases of mental and physical problems.
“If you think of the brain as an extremely complex machine, how could we understand it without first breaking it down and knowing the parts?” asked cellular neuroscientist Helen Bateup, a University of California, Berkeley, associate professor of molecular and cell biology and co-author of the flagship paper that synthesizes the results of the other papers. “The first page of any manual of how the brain works should read: Here are all the cellular components, this is how many of them there are, here is where they are located and who they connect to.”
Individual researchers have previously identified dozens of cell types based on their shape, size, electrical properties and which genes are expressed in them. The new studies identify about five times more cell types, though many are subtypes of well-known cell types. For example, cells that release specific neurotransmitters, like gamma-aminobutyric acid (GABA) or glutamate, each have more than a dozen subtypes distinguishable from one another by their gene expression and electrical firing patterns.
While the current papers address only the motor cortex, the BRAIN Initiative Cell Census Network (BICCN) — created in 2017 — endeavors to map all the different cell types throughout the brain, which consists of more than 160 billion individual cells, both neurons and support cells called glia. The BRAIN Initiative was launched in 2013 by then-President Barack Obama.
“Once we have all those parts defined, we can then go up a level and start to understand how those parts work together, how they form a functional circuit, how that ultimately gives rise to perceptions and behavior and much more complex things,” Bateup said.
Together with former UC Berkeley professor John Ngai, Bateup and UC Berkeley colleague Dirk Hockemeyer have already used CRISPR-Cas9 to create mice in which a specific cell type is labeled with a fluorescent marker, allowing them to track the connections these cells make throughout the brain. For the flagship journal paper, the Berkeley team created two strains of “knock-in” reporter mice that provided novel tools for illuminating the connections of the newly identified cell types, she said.
“One of our many limitations in developing effective therapies for human brain disorders is that we just don’t know enough about which cells and connections are being affected by a particular disease and therefore can’t pinpoint with precision what and where we need to target,” said Ngai, who led UC Berkeley’s Brain Initiative efforts before being tapped last year to direct the entire national initiative. “Detailed information about the types of cells that make up the brain and their properties will ultimately enable the development of new therapies for neurologic and neuropsychiatric diseases.”
Ngai is one of 13 corresponding authors of the flagship paper, which has more than 250 co-authors in all.
Bateup, Hockemeyer and Ngai collaborated on an earlier study to profile all the active genes in single dopamine-producing cells in the mouse’s midbrain, which has structures similar to human brains. This same profiling technique, which involves identifying all the specific messenger RNA molecules and their levels in each cell, was employed by other BICCN researchers to profile cells in the motor cortex. This type of analysis, using a technique called single-cell RNA sequencing, or scRNA-seq, is referred to as transcriptomics.
The scRNA-seq technique was one of nearly a dozen separate experimental methods used by the BICCN team to characterize the different cell types in three different mammals: mice, marmosets and humans. Four of these involved different ways of identifying gene expression levels and determining the genome’s chromatin architecture and DNA methylation status, which is called the epigenome. Other techniques included classical electrophysiological patch clamp recordings to distinguish cells by how they fire action potentials, categorizing cells by shape, determining their connectivity, and looking at where the cells are spatially located within the brain. Several of these used machine learning or artificial intelligence to distinguish cell types.
“This was the most comprehensive description of these cell types, and with high resolution and different methodologies,” Hockemeyer said. “The conclusion of the paper is that there’s remarkable overlap and consistency in determining cell types with these different methods.”
A team of statisticians combined data from all these experimental methods to determine how best to classify or cluster cells into different types and, presumably, different functions based on the observed differences in expression and epigenetic profiles among these cells. While there are many statistical algorithms for analyzing such data and identifying clusters, the challenge was to determine which clusters were truly different from one another — truly different cell types — said Sandrine Dudoit, a UC Berkeley professor and chair of the Department of Statistics. She and biostatistician Elizabeth Purdom, UC Berkeley associate professor of statistics, were key members of the statistical team and co-authors of the flagship paper.
“The idea is not to create yet another new clustering method, but to find ways of leveraging the strengths of different methods and combining methods and to assess the stability of the results, the reproducibility of the clusters you get,” Dudoit said. “That’s really a key message about all these studies that look for novel cell types or novel categories of cells: No matter what algorithm you try, you’ll get clusters, so it is key to really have confidence in your results.”
Bateup noted that the number of individual cell types identified in the new study depended on the technique used and ranged from dozens to 116. One finding, for example, was that humans have about twice as many different types of inhibitory neurons as excitatory neurons in this region of the brain, while mice have five times as many.
“Before, we had something like 10 or 20 different cell types that had been defined, but we had no idea if the cells we were defining by their patterns of gene expression were the same ones as those defined based on their electrophysiological properties, or the same as the neuron types defined by their morphology,” Bateup said.
“The big advance by the BICCN is that we combined many different ways of defining a cell type and integrated them to come up with a consensus taxonomy that’s not just based on gene expression or on physiology or morphology, but takes all of those properties into account,” Hockemeyer said. “So, now we can say this particular cell type expresses these genes, has this morphology, has these physiological properties, and is located in this particular region of the cortex. So, you have a much deeper, granular understanding of what that cell type is and its basic properties.”
Dudoit cautioned that future studies could show that the number of cell types identified in the motor cortex is an overestimate, but the current studies are a good start in assembling a cell atlas of the whole brain.
“Even among biologists, there are vastly different opinions as to how much resolution you should have for these systems, whether there is this very, very fine clustering structure or whether you really have higher level cell types that are more stable,” she said. “Nevertheless, these results show the power of collaboration and pulling together efforts across different groups. We’re starting with a biological question, but a biologist alone could not have solved that problem. To address a big challenging problem like that, you want a team of experts in a bunch of different disciplines that are able to communicate well and work well with each other.”
Other members of the UC Berkeley team included postdoctoral scientists Rebecca Chance and David Stafford, graduate student Daniel Kramer, research technician Shona Allen of the Department of Molecular and Cell Biology, doctoral student Hector Roux de Bézieux of the School of Public Health and postdoctoral fellow Koen Van den Berge of the Department of Statistics. Bateup is a member of the Helen Wills Neuroscience Institute, Hockemeyer is a member of the Innovative Genomics Institute, and both are investigators funded by the Chan Zuckerberg Biohub.
TOP SCEINCE
New drug shows promise in clearing HIV from brain
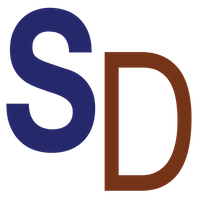
An experimental drug originally developed to treat cancer may help clear HIV from infected cells in the brain, according to a new Tulane University study.
Published in the journal Brain, this discovery marks a significant step toward eliminating HIV from hard-to-reach reservoirs where the virus evades otherwise effective treatment.
“This research is an important step in tackling brain-related issues caused by HIV, which still affect people even when they are on effective HIV medication,” said lead study author Woong-Ki Kim, PhD, associate director for research at Tulane National Primate Research Center. “By specifically targeting the infected cells in the brain, we may be able to clear the virus from these hidden areas, which has been a major challenge in HIV treatment.”
Antiretroviral therapy (ART) is an essential component of successful HIV treatment, maintaining the virus at undetectable levels in the blood and transforming HIV from a terminal illness into a manageable condition. However, ART does not completely eradicate HIV, necessitating lifelong treatment. The virus persists in “viral reservoirs” in the brain, liver, and lymph nodes, where it remains out of reach of ART.
The brain has been a particularly challenging area for treatment due to the blood-brain barrier — a protective membrane that shields it from harmful substances but also blocks treatments, allowing the virus to persist. In addition, cells in the brain known as macrophages are extremely long-lived, making them difficult to eradicate once they become infected.
Infection of macrophages is thought to contribute to neurocognitive dysfunction, experienced by nearly half of those living with HIV. Eradicating the virus from the brain is critical for comprehensive HIV treatment and could significantly improve the quality of life for those with HIV-related neurocognitive problems.
Researchers focused on macrophages, a type of white blood cell that harbors HIV in the brain. By using a small molecule inhibitor to block a receptor that increases in HIV-infected macrophages, the team successfully reduced the viral load in the brain. This approach essentially cleared the virus from brain tissue, providing a potential new treatment avenue for HIV.
The small molecule inhibitor used, BLZ945, has previously been studied for therapeutic use in amyotrophic lateral sclerosis (ALS) and brain cancer, but never before in the context of clearing HIV from the brain.
The study, which took place at the Tulane National Primate Research Center, utilized three groups to model human HIV infection and treatment: an untreated control group, and two groups treated with either a low or high dose of the small molecule inhibitor for 30 days. The high-dose treatment lead to a notable reduction in cells expressing HIV receptor sites, as well as a 95-99% decrease in viral DNA loads in the brain .
In addition to reducing viral loads, the treatment did not significantly impact microglia, the brain’s resident immune cells, which are essential for maintaining a healthy neuroimmune environment. It also did not show signs of liver toxicity at the doses tested.
The next step for the research team is to test this therapy in conjunction with ART to assess its efficacy in a combined treatment approach. This could pave the way for more comprehensive strategies to eradicate HIV from the body entirely.
This research was funded by the National Institutes of Health, including grants from the National Institute of Mental Health and the National Institute of Neurological Disorders and Stroke, and was supported with resources from the Tulane National Primate Research Center base grant of the National Institutes of Health, P51 OD011104.
TOP SCEINCE
Chemical analyses find hidden elements from renaissance astronomer Tycho Brahe’s alchemy laboratory
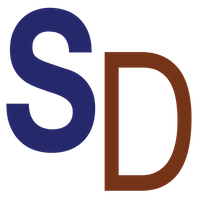
In the Middle Ages, alchemists were notoriously secretive and didn’t share their knowledge with others. Danish Tycho Brahe was no exception. Consequently, we don’t know precisely what he did in the alchemical laboratory located beneath his combined residence and observatory, Uraniborg, on the now Swedish island of Ven.
However, during an excavation in 1988-1990, some pottery and glass shards were found in Uraniborg’s old garden. These shards were believed to originate from the basement’s alchemical laboratory. Five of these shards — four glass and one ceramic — have now undergone chemical analyses to determine which elements the original glass and ceramic containers came into contact with.
The chemical analyses were conducted by Professor Emeritus and expert in archaeometry, Kaare Lund Rasmussen from the Department of Physics, Chemistry, and Pharmacy, University of Southern Denmark. Senior researcher and museum curator Poul Grinder-Hansen from the National Museum of Denmark oversaw the insertion of the analyses into historical context.
Enriched levels of trace elements were found on four of them, while one glass shard showed no specific enrichments. The study has been published in the journal Heritage Science.
“Most intriguing are the elements found in higher concentrations than expected — indicating enrichment and providing insight into the substances used in Tycho Brahe’s alchemical laboratory,” said Kaare Lund Rasmussen.
The enriched elements are nickel, copper, zinc, tin, antimony, tungsten, gold, mercury, and lead, and they have been found on either the inside or outside of the shards.
Most of them are not surprising for an alchemist’s laboratory. Gold and mercury were — at least among the upper echelons of society — commonly known and used against a wide range of diseases.
“But tungsten is very mysterious. Tungsten had not even been described at that time, so what should we infer from its presence on a shard from Tycho Brahe’s alchemy workshop?,” said Kaare Lund Rasmussen.
Tungsten was first described and produced in pure form more than 180 years later by the Swedish chemist Carl Wilhelm Scheele. Tungsten occurs naturally in certain minerals, and perhaps the element found its way to Tycho Brahe’s laboratory through one of these minerals. In the laboratory, the mineral might have undergone some processing that separated the tungsten, without Tycho Brahe ever realizing it.
However, there is also another possibility that Professor Kaare Lund Rasmussen emphasizes has no evidence whatsoever — but which could be plausible.
Already in the first half of the 1500s, the German mineralogist Georgius Agricola described something strange in tin ore from Saxony, which caused problems when he tried to smelt tin. Agricola called this strange substance in the tin ore “Wolfram” (German for Wolf’s froth, later renamed to tungsten in English).
“Maybe Tycho Brahe had heard about this and thus knew of tungsten’s existence. But this is not something we know or can say based on the analyses I have done. It is merely a possible theoretical explanation for why we find tungsten in the samples,” said Kaare Lund Rasmussen.
Tycho Brahe belonged to the branch of alchemists who, inspired by the German physician Paracelsus, tried to develop medicine for various diseases of the time: plague, syphilis, leprosy, fever, stomach aches, etc. But he distanced himself from the branch that tried to create gold from less valuable minerals and metals.
In line with the other medical alchemists of the time, he kept his recipes close to his chest and shared them only with a few selected individuals, such as his patron, Emperor Rudolph II, who allegedly received Tycho Brahe’s prescriptions for plague medicine.
We know that Tycho Brahe’s plague medicine was complicated to produce. It contained theriac, which was one of the standard remedies for almost everything at the time and could have up to 60 ingredients, including snake flesh and opium. It also contained copper or iron vitriol (sulphates), various oils, and herbs.
After various filtrations and distillations, the first of Brahe’s three recipes against plague was obtained. This could be made even more potent by adding tinctures of, for example, coral, sapphires, hyacinths, or potable gold.
“It may seem strange that Tycho Brahe was involved in both astronomy and alchemy, but when one understands his worldview, it makes sense. He believed that there were obvious connections between the heavenly bodies, earthly substances, and the body’s organs. Thus, the Sun, gold, and the heart were connected, and the same applied to the Moon, silver, and the brain; Jupiter, tin, and the liver; Venus, copper, and the kidneys; Saturn, lead, and the spleen; Mars, iron, and the gallbladder; and Mercury, mercury, and the lungs. Minerals and gemstones could also be linked to this system, so emeralds, for example, belonged to Mercury,” explained Poul Grinder-Hansen.
Kaare Lund Rasmussen has previously analyzed hair and bones from Tycho Brahe and found, among other elements, gold. This could indicate that Tycho Brahe himself had taken medicine that contained potable gold.
TOP SCEINCE
Nitrogen emissions have a net cooling effect: But researchers warn against a climate solution
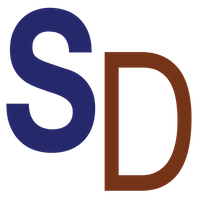
An international team of researchers has found that nitrogen emissions from fertilisers and fossil fuels have a net cooling effect on the climate. But they warn increasing atmospheric nitrogen has further damaging effects on the environment, calling for an urgent reduction in greenhouse gas emissions to halt global warming.
The paper was led by the Max Planck Institute in Germany and included authors from the University of Sydney. It comes one day after new data from the European Union’s Copernicus Climate Change Service indicated that Sunday, 21 July was the hottest day recorded in recent history.
The net cooling effect occurs in four ways:
- Short-lived nitrogen oxides produced by the combustion of fossil fuels pollute the atmosphere by forming fine suspended particles which shield sunlight, in turn cooling the climate;
-
ammonia (a nitrogen and hydrogen-based compound) released into the atmosphere from the application of manure and artificial fertilisers has a similar effect;
-
nitrogen applied to crops allows plants to grow more abundantly, absorbing more CO2 from the atmosphere, enabling a cooling effect;
-
nitrogen oxides also play a role in the breakdown of atmospheric methane, a potent greenhouse gas.
The researchers warned that increasing atmospheric nitrogen was not a solution for combatting climate change.
“Nitrogen fertilisers pollute water and nitrogen oxides from fossil fuels pollute the air. Therefore, increasing rates of nitrogen in the atmosphere to combat climate change is not an acceptable compromise, nor is it a solution,” said Professor Federico Maggi from the University of Sydney’s School of Civil Engineering.
Sönke Zaehle from the Max Planck Institute said: “This may sound like good news, but you have to bear in mind that nitrogen emissions have many harmful effects, for example on health, biodiversity and the ozone layer. The current findings, therefore, are no reason to gloss over the harmful effects, let alone see additional nitrogen input as a means of combatting global warming.”
Elemental nitrogen, which makes up around 78 percent of the air, is climate-neutral, but other reactive nitrogen compounds can have direct or indirect effects on the global climate — sometimes warming and at other times cooling. Nitrous oxide (N2O) is an almost 300 times more potent greenhouse gas than CO2. Other forms of nitrogen stimulate the formation of ozone in the troposphere, which is a potent greenhouse gas and enhances global warming.
Professor Maggi said the research was important as it helped the team gain an understanding of the net-effect of the distribution of nitrogen emissions from agriculture.
“This work is an extraordinary example of how complex interactions at planetary scales cannot be captured with simplistic assessment tools. It shows the importance of developing mathematical models that can show the emergence of nonlinear — or unproportional — effects across soil, land, and atmosphere,” he said.
“Even if it appears counter-intuitive, reactive nitrogen introduced in the environment, mostly as agricultural fertilisers, can reduce total warming. However, this is minor compared with the reduction in greenhouse gas emissions required to keep the planet within safe and just operational boundaries.
“New generation computational tools are helping drive new learnings in climate change science, but understanding is not enough — we must act with great urgency to reduce greenhouse gas emissions.”
Gaining a holistic understanding of the impacts of nitrogen
The scientists determined the overall impact of nitrogen from human sources by first analysing the quantities of the various nitrogen compounds that end up in soil, water and air.
They then fed this data into models that depict the global nitrogen cycle and the effects on the carbon cycle, for example the stimulation of plant growth and ultimately the CO2 and methane content of the atmosphere. From the results of these simulations, they used another atmospheric chemistry model to calculate the effect of man-made nitrogen emissions on radiative forcing, that is the radiant energy that hits one square metre of the Earth’s surface per unit of time.
-
Solar Energy3 years ago
DLR testing the use of molten salt in a solar power plant in Portugal
-
Camera3 years ago
Charles ‘Chuck’ Geschke, co-founder of Adobe and inventor of the PDF, dies at 81
-
world news8 months ago
Gulf, France aid Gaza, Russia evacuates citizens
-
world news3 months ago
Jewish diaspora expresses concern as Iranian drones launch toward Israel
-
Camera3 years ago
80,000MP panoramas: EarthCam announces world’s highest-resolution robotic webcam
-
TOP SCEINCE3 months ago
Can animals count?
-
Solar Energy8 months ago
Glencore eyes options on battery recycling project
-
Camera8 months ago
DJI Air 3 vs. Mini 4 Pro: which compact drone is best?